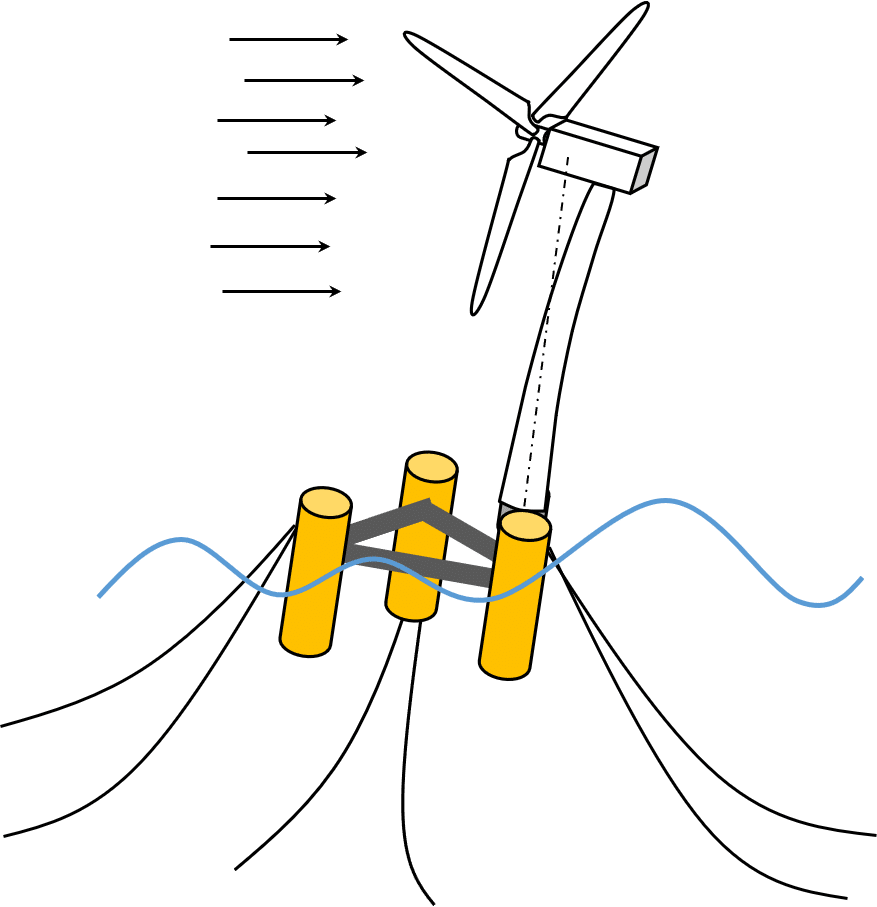
We build digital twins of wind turbines, to enable model-based applications, like structural load monitoring, mooring integrity and fatigue monitoring or predictive maintenance. Our SLOW (Simplified Low-Order Wind turbine) software is the base of the digital twin and together with Kalman filters we feed in sensor signals to update to measurements and the reality.
For floating wind turbine monitoring we have partnered with AMOG Engineering. Read more about our joint product on www.fowt-monitoring.com
Step by step
Pre-FEED FOWT design for ULS and FLS
Tower
Mooring lines
Platform watch circle
Aero-Hydro-Servo-Elastic Response Amplitude Operator (RAO)
Annual Energy Production (AEP)
Extensive sensitivity studies and design optimization
Controller design
Real-time digital twin
Online simulation, fed by sensor data for load prediction and uncertainty estimate
State-observer calculating the best estimate of loads, merging sensor and model information; Accurate data source for fatigue and Condition Monitoring [3]
Our Approach
- Structural dynamics: 2D and 3D rigid- or flexible-body motion, aligned or misaligned wind and waves. Platform rigid-body motion, elastic tower deformation, rigid rotor
- Aerodynamics: Actuator-disk model of the lumped rotor,
wind misalignment by cosine-portion of aerodynamic forces - Hydrodynamics: Linear potential flow hydrodynamics, with
simplified radiation model, augmented by viscous drag from
Morison’s equation - Mooring dynamics: Quasi-static or dynamic nonlinear model
- Controller dynamics: Blade pitch, generator torque
- Integrator: Runge-Kutta 4th order
- Architecture: Portable executable, text-based input files
(yaml format)
Extensions
- Dynamic mooring model for mooring fatigue assessment
- Blade structural dynamics model (as in OrcaFlex, OpenFAST, Bladed) to resolve blade loads and Three-Times-Per-Revolution (3p) tower loads
- Blade-Element Momentum theory (as in OrcaFlex, OpenFAST, Bladed) to analyze misaligned inflow and wind shear influence
- Elastic floater beam model for a better approximation of global tower natural frequency and the possibility to extract internal floater loads for hull design
Usage
- User guide: Manual with easy-to-use tutorials
- Controller: Bladed-style Dynamic Link Library (DLL)
- Batch runs: Easy parallelization by user due to portable executable without installation (MS Windows)
- Postprocessing: Files readable by PyDatView
- Structural design: Output signals for
- Hydrostatic design (trim in operation/idling)
- Mooring design (fairlead tensions, watch circle)
- Tower design (tower-base section loads)
- Controller design (rotor speed, generator torque, blade pitch angle, el. power, shaft loads)
References and Track Record
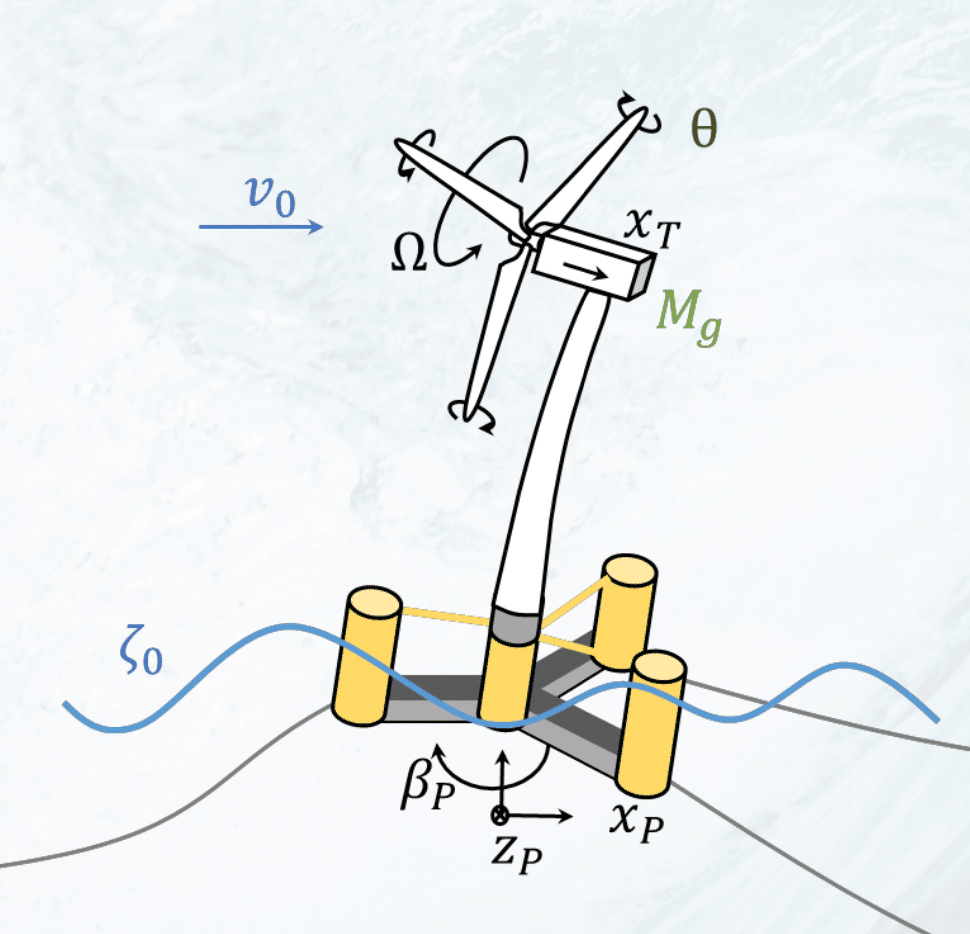
- SLOW has been used in numerous FOWT design and controller design projects
- SLOW has been extensively compared against OpenFAST, i.e. [1]
- SLOW has been validated against two scaled experiments [2,3]
[1] Lemmer, F., Yu, W., Luhmann, B., Schlipf, D., & Cheng, P. W. (2020). Multibody modeling for concept-level floating offshore wind turbine design. Multibody System Dynamics, 49(2), 203–236. https://doi.org/10.1007/s11044-020-09729-x
[2] Lemmer, F., Yu, W., Cheng, P. W., Pegalajar-Jurado, A., Borg, M., Mikkelsen, R., & Bredmose, H. (2018). The TripleSpar campaign: Validation of a reduced-order simulation model for floating wind turbines. Proceedings of the ASME 37th International Conference on Ocean, Offshore and Arctic Engineering. https://doi.org/10.1115/OMAE2018-78119
[3] Lemmer, F., Lehmann, K., Raach, S., Al, M., Skandali, D., Schlipf, D., … Cheng, P. W. (2021). Assessment of a state-feedback controller and observer in a Floating Wind scaled experiment. Proceedings of the Wind Energy Science Conference. https://doi.org/10.5281/zenodo.5004916
[4] Lemmer, F., Yu, W., Schlipf, D., & Cheng, P. W. (2020). Robust gain scheduling baseline controller for floating offshore wind turbines. Wind Energy, 23(1). https://doi.org/10.1002/we.2408
[5] Lemmer, F., Schlipf, D., & Cheng, P. W. (2016). Control design methods for floating wind turbines for optimal disturbance rejection. Journal of Physics: Conference Series, 753. https://doi.org/10.18419/opus-8906
[6] Schlipf, D., Lemmer, F., & Raach, S. (2020). Multi-variable feedforward control for floating wind turbines using lidar. Proceedings of the 18th International Offshore and Polar Engineering Conference. https://doi.org/10.18419/opus-11067
[7] Schlipf, D., Sandner, F., Raach, S., Matha, D., & Cheng, P. W. (2013). Nonlinear model predictive control of floating wind turbines. Proceedings of the 23rd International Ocean and Polar Engineering Conference, 440–447. https://doi.org/10.18419/opus-3908